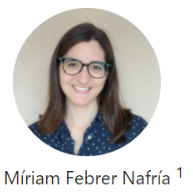
1 Postdoctoral Researcher, Biomechanical Engineering Lab (BIOMEC), Diagonal 647, 08028 Barcelona, Spain.
Biomechanics of human movement and predictive simulations
Biomechanics of human movement is the science that studies human locomotion, i.e., how and why do we move. Understanding better how movement is produced, can help us to find new treatments, to define novel rehabilitation plans or to design personalised assistive devices to improve the mobility of people with skeletal and neuro-muscular disorders [Uchida and Delp, 2021]. This can be done through motion simulation using mathematical models that represent the human neuro-musculoskeletal system [De Groote and Falisse, 2021]. The human neuro-musculoskeletal system can be viewed as a servo-controlled multibody dynamic system, where bones are modelled as rigid bodies connected by joints, actuated by muscles and controlled by the central nervous system. A group of bones is usually represented as a single rigid body if there is no relative motion between them or it is not relevant for the purpose of the study (e.g., motion of hand or fingers in walking). Synovial and cartilaginous joints, that are the joints that permit relative motion between segments, can be modelled as mechanical joints. For instance, shoulder and hip are usually defined as spherical joints, while knee is usually represented as a revolute joint, although more complex models allowing 3D rotations and 3D translations have also been used. Skeletal muscles, which are the ones that link to bones through tendons and cause motion, are usually modelled using a Hill-type muscle model. In these models, muscles and tendons are modelled as a linear actuator, which exhibits an active behaviour, produced by the muscle fibres, and a passive elastic behaviour, related to the tendons and the connective tissue wrapping the muscle fibres.
Computer simulations based on multibody dynamics can be used to analyse human movements and calculate quantities that cannot be (easily) directly measured (e.g., muscle forces or joint contact forces). We can distinguish between simulations that analyse a known movement (i.e., motion or forces are known, because they have been measured), and simulations that predict new movements, for which experimental data are unknown. Typically, a dynamic analysis starts collecting experimental motion data to compute joint angle time histories [Uchida and Delp, 2021]. Knowing the evolution of these coordinates and the motion equations of the human body model allows for calculating the net joint torques that produce that movement. This first type of analysis, from motion to forces, is called inverse dynamic analysis and its solution is unique based on the mechanical laws of motion. However, once forces and torques are known for each joint, there are multiple solutions of muscle forces that yield that unique joint torque, because the neuro-musculoskeletal model is an over-actuated system (i.e., each joint is actuated by different muscles. This is called the muscle force redundancy problem (or muscle force-sharing problem). This problem is usually solved using optimisation procedures based on physiological criteria, where a cost function is minimised. That cost function aims to represent the neural control strategy, used by the central nervous system, to coordinate muscle activation for a certain motion task. On the other hand, if we start from forces and torques and we want to obtain the motion that they produce, the motion ordinary differential equations have to be integrated with respect to time. This approach is known as forward dynamic analysis, and allows to do research on how motion is affected when force time histories or certain model parameters are varied.
If a new movement (for which no experimental data have been collected) has to be predicted, the most accepted approach consists of formulating an optimisation problem. It is believed that there is an optimal principle that guides human motion, although it is not clearly known which is the cost function that mathematically represents it. Predictive simulations can help us to find these principles, by comparing the results of simulations that minimise different magnitudes with experimental motion [De Groote and Falisse, 2021]. Generally, the cost function is assumed a priori, based on previous published studies or some assumptions. It has been found that minimising metabolic energy cost and minimising muscle activation produce realistic gait patterns, and that the cost function may include different criteria, as the use of multiple criteria leads to improved results. Nowadays, direct collocation optimal control is the most widespread optimisation method for developing predictive simulations of human motion [De Groote and Falisse, 2021]. Predicting novel motions can help to anticipate the result of surgery, to design assistive devices, such as prosthetics and orthoses, to develop rehabilitation therapies, and to learn how an exercise should be performed in an optimal way. Neuro-musculoskeletal modelling and optimal control are two fields that have recently been related for designing patient-specific treatments to improve walking function in subjects affected by neuro-musculoskeletal impairments. However, more research is needed to improve the process of creating and validating movement simulations of individuals with impairments, before they can be used in real clinical practice [Fregly, 2021].
Case study: Personalisation of active orthosis actuation parameters for spinal cord-injured subjects
Each year, between 250,000 and 500,000 people worldwide suffer a spinal cord injury. Walking impairment after spinal cord injury leads to a decreased quality of life, other serious health conditions and substantial health care costs. Consequently, gait restoration is a high priority among spinal cord-injured (SCI) subjects; and it can be partially achieved using active orthoses or exoskeletons, together with some type of external support for balance (e.g., crutches or a walker). Customisation of active orthoses control algorithms to maximise the walking ability of each patient is currently done through trial-and-error methods, making it difficult if not impossible to identify the best active control parameters for any particular patient. To improve the walking ability of each patient as much as possible, it is important to personalise conveniently the parameters that define the device actuation, which may be different for each subject. We believe that this personalisation could be done using an optimal control predictive approach, thus avoiding (or reducing) the experimental trial-and-error process of manually adjusting these actuation parameters for each patient.
Before developing a prediction framework to predict crutch-orthosis-assisted walking, a first stepping stone is to predict crutch-assisted walking patterns. To date, crutch walking simulation studies have been performed using simple models and without focusing on specific clinical applications. We developed an optimal control problem formulation capable of predicting different patterns of crutch-assisted walking, using a 3D torque-driven full-body human model that includes arms and crutches [Febrer-Nafría et al., 2021]. Then, we investigated whether the use of a computational approach (optimal control prediction) to personalise pre-defined knee actuation parameters for an active knee-ankle-foot orthosis (KAFO) [Font-Llagunes et al., 2020] would be a better choice than the current trial-and-error approach. We explored different optimal control problem formulations that allowed to simulate different pre-defined assistive knee angle trajectories, so that the best walking pattern for a specific individual with spinal cord injury could be identified [Febrer-Nafría et al., 2022].
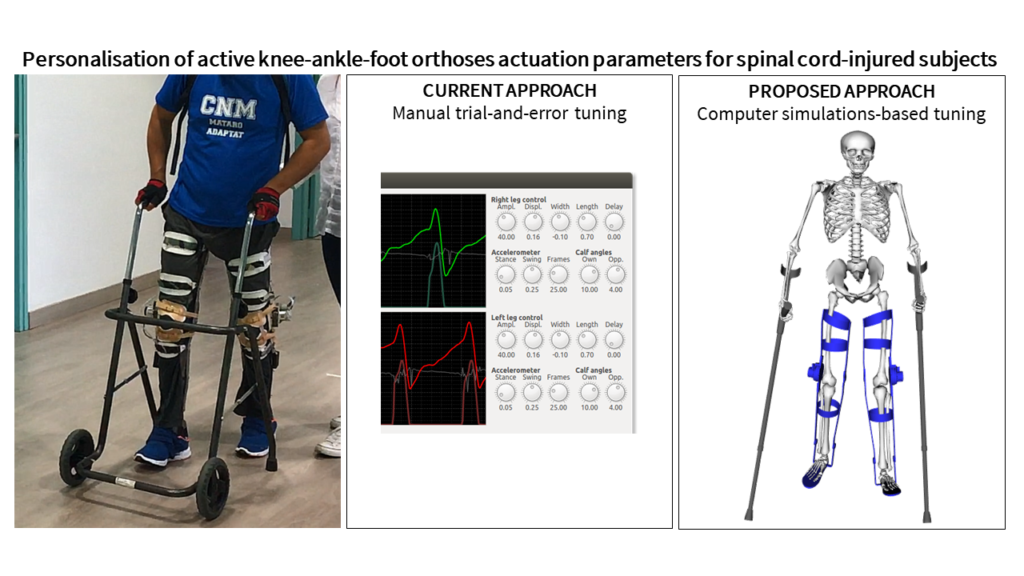
Our study did not compare directly the simulation approach with manual tuning, but rather explored optimal control problem formulations that allowed different pre-defined assistive knee angle trajectories to be simulated, thereby permitting identification of the best walking pattern for a specific individual with SCI. This goal was pursued by using a new optimal control problem formulation for predicting crutch-orthosis-assisted walking of an SCI subject wearing the presented active orthoses, given as an initial guess the subject’s gait without knee flexion-extension assistance (i.e., locked knee) and imposing a specific pre-defined knee angle trajectory. In this work, the maximum knee flexion angle parameter, which is usually the first one tuned in the trial-and-error approach, was investigated. Given the subject’s gait with passive walking aids, different knee trajectories (with four different maximum knee flexion angle parameters) were tested computationally. Improvements in gait pattern were quantified in terms of changes in clinically meaningful/relevant measurements (foot clearance, stride length, cadence, and hip flexion ROM) with respect to predicted locked knee motion. The best parameter values were found for moderate knee flexion.
Although more research is needed before this method can be applied to choose optimal active orthosis actuation parameters for a specific subject, these findings represent a step forward in the computational personalisation of predefined knee angle trajectories for the control of an active KAFO for SCI subjects. We consider that having a simulation tool that allows testing of different pre-defined knee motions for a specific SCI subject model, with the aim of finding a more balanced and improved assisted gait pattern, will overcome the limitations of manual personalization of pre-specified knee motion parameter values and will result in an improved assisted motion for each SCI subject.
References
De Groote, F., & Falisse, A. (2021). Perspective on musculoskeletal modelling and predictive simulations of human movement to assess the neuromechanics of gait. Proceedings of the Royal Society B, 288(1946), 20202432.
Febrer-Nafría, M., Pallarès-López, R., Fregly, B. J., & Font-Llagunes, J. M. (2021). Prediction of three-dimensional crutch walking patterns using a torque-driven model. Multibody System Dynamics, 51(1), 1-19.
Febrer-Nafría, M., Fregly, B. J., & Font-Llagunes, J. M. (2022). Evaluation of Optimal Control Approaches for Predicting Active Knee-Ankle-Foot-Orthosis Motion for Individuals With Spinal Cord Injury. Frontiers in Neurorobotics, 15.
Font-Llagunes, J. M., Lugrís, U., Clos, D., Alonso, F. J., & Cuadrado, J. (2020). Design, control, and pilot study of a lightweight and modular robotic exoskeleton for walking assistance after spinal cord injury. Journal of Mechanisms and Robotics, 12(3), 031008.
Fregly, B. J. (2021). A conceptual blueprint for making neuromusculoskeletal models clinically useful. Applied Sciences, 11(5), 2037.
Uchida, T. K., & Delp, S. L. (2021). Biomechanics of movement: the science of sports, robotics, and rehabilitation. MIT Press.